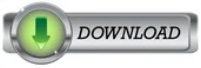

In the present study, a computational screening protocol combining DFT, scaling relations, and microkinetic modeling allows for a rational selection of novel catalysts that can deliver efficient glycerol electrooxidation, low cost of production, and environmental sustainability. The development of novel and efficient catalytic electrode materials for the anodic side of the reaction is a key towards a hydrogen-based energy economy. By means of electrooxidation, glycerol can be used as a feedstock for scalable hydrogen production, in addition to conversion to value-added products. Glycerol is a byproduct of biodiesel production and, as such, it is of limited economic value. Moreover, the intrinsic size- and metal-dependent activity are analyzed on the basis of dominant reaction pathway, which are fundamentally related to C-metal and O-metal binding ability, respectively. In addition, the metal-dependent activity is elucidated, with the activity following Rh > Ni > Pd ~ Pt. M(211) is the active surface, and the increased M(211) surface fraction with decreasing particle size results in enhanced activity. It is found that activity is inversely proportional to particle size, and this size-dependent activity results from the fraction change in surfaces with distinct activity. Herein, we report an approach that combining microkinetic modeling with a truncated octahedron model to explore the size- and metal-dependent activity, using steam methane reforming on metallic (M= Rh, Ni, Pt and Pd) nanoparticles as a model system. Systematical and in-depth understanding of size- and metal-dependent activity plays a pivotal role in nanocatalysis for rational design of highly efficient catalysts. We propose a way to overcome the computational challenges, that can be applied to simulations corresponding to doped semiconductors in general. This points towards a computational challenge in describing the doped oxides in a realistic manner. Our analysis suggested that trends in doped semiconducting oxides behave very differently from the other oxides. On the other hand, the data did not show clear trend line regarding the O∗ binding. The data revealed that the universal scaling relation between HO∗ and HOO∗ intermediates is still present and robust, despite the variety in methods and structures used for calculating the binding energies of the intermediates. In the present work, we collected published studies involving DFT calculations for the OER, with the purpose to understand why the progress made so far, for lowering the overpotential of the reaction, is relatively small. Despite the substantial efforts, the driving force required for water oxidation is largely making the reaction inefficient. Multiple strategies to overcome the intrinsic limitations of the oxygen evolution reaction (OER) have been conducted by numerous research groups. In situ/operando X-ray absorption spectroscopy analyses further corroborate the importance of reduced Ni⁺ species in boosting the CO2RR activity. DFT calculations combined with micro-kinetic modeling suggest that the Ni–N3V site appears to be responsible for the high CO2RR activity because of its lower barrier for the formation of *COOH intermediate and optimum *CO binding energy. Extended X-ray absorption fine structure analysis and density functional theory (DFT) calculations reveal that the heat treatment transforms molecular Ni²⁺–N4 sites of NiPc into Ni⁺–N3V (V: vacancy) and Ni⁺–N3 sites incorporated in the graphene lattice that concomitantly involves a breakage of Ni–N bonding, shrinkage in the Ni–N–C local structure, and decrease in the oxidation state of the Ni center from +2 to +1. H-NiPc/CNT exhibits ~4.7-fold higher turnover frequency for CO2RR to CO in comparison to NiPc/CNT. In this work, Ni(II) phthalocyanine molecules grafted on carbon nanotube (NiPc/CNT) and heat-treated NiPc/CNT (H-NiPc/CNT) are exploited as model catalysts to investigate the impact of thermal activation on the structure of active site and CO2RR activity. However, the high-temperature treatment typically involved during the activation of Ni–N/C catalysts makes the origin of the high activity elusive. Atomically dispersed nickel sites complexed on nitrogen-doped carbon (Ni–N/C) have demonstrated considerable activity for the selective electrochemical carbon dioxide reduction reaction (CO2RR) to CO.
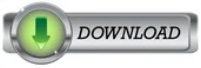